CRISPR-Cas9 is the hallmark of bioinformatics and genetics and has revolutionized genome editing by analyzing biomarkers in certain nucleotide bases that cause mutations and lead to different diseases. CRISPR-Cas9 is a popular multi complex technological tool that stands for “Clustered Regularly Interspaced Short Palindromic Repeats” 1 The discovery of this system came from looking at the bacterial genome of E.coli, containing palindromic repeats, becoming an important standpoint for “adaptive prokaryotic immune system mechanisms”. 2 This new technology provides a reliable and precise method of genetic manipulation of DNA. Genome editing is performed by specific nucleases, creating regions of the genome that induce double-stranded breaks. 3
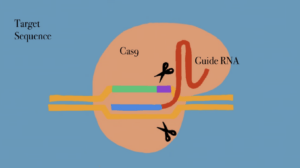
The mechanism used for this repair is derived from non-homologous end-joining (NHEJ), however, it is prone to error. Nonetheless, it also protects chromosomes from degradation and prevents nucleotide deletions and insertions. The system entails improvements in surrounding the applications of gene therapy, transcription regulation, disease tracking and images of nuclease domains 4 . Because of all the different reasons exposed above, there is no doubt that CRISPR has the ability to improve people’s lives, but there are other factors to consider. For instance, there are many ethical issues that arise when CRISPR is discussed, including doubts regarding the precision of the technology, concerns involving the long-term generational effects of DNA editing in germ line cells, and how CRISPR will impact people’s medical decisions.
Genome editing technologies are informative and known for their use of precision and accuracy in repair mechanisms involving DNA. Just as this technology could be incredibly helpful in different medical situations, it could also have catastrophic consequences. Like everything in this world, there are always factors of error and, since we are talking about human life and its future, safety concerns regarding the CRISPR-Cas9 technology system are brought up by the bioethical community. For that reason, CRISPR-Cas9 safety in human health became an important topic in research applications involving off-target activity due to random errors and alterations in the genomic DNA. Off-target effects could occur any time CRISPR makes a mistake while performing its function, which would later lead to a genetic change that was unforeseen. 5 Of course, this unpredicted genetic change could be good, but what happens if it is not? The development of new diseases could occur and those would be the new problems in the need of a solution.
An example is the study conducted by Zuccaro et al. (2020) on Allele-Specific Chromosome Removal after Cas9 Cleavage in Human Embryos. 6 To analyze deletions induced by Cas9, the researchers of the previously mentioned study looked at double stranded breaks due to them having hidden recombination. Scientists recruited patients that accompanied the requirements of loss of vision and homozygosity for the G deletion mutation in the EYS gene. 5 These requirements were established in order to determine the gRNA for the allele that contained the mutation and repair Cas9’s influence on double stranded breaks in DNA on the paternal chromosome for the EYS locus. The EYS encodes a frameshift mutation which causes substantial changes through an addition or deletion of a nucleotide changing the reading frame. 5
This will result in blindness of the individual with that particular locus on the paternal chromosome. The mutation occurs in the zygote and leads to “non-mosaic restoration of the reading frame”.5 This indicates that when the DNA is broken, only 50% is unrepaired, which results in the deletion of information from the paternal allele, making it unrecognizable. 5 The repair mechanism used for this mutation resulted in non-homologous end-joining which is prone to error, however, it mediates chromosomes for degradation of RNA digestive enzymes.
Cas9 influenced Off-target cleavage to show chromosomal loss and indels. This led to cautions using Cas9 induced off and on targeting activity since it caused an abnormal number of chromosomes to be present in the organism. 5 This introduces the bioethical concern for long term generational effects due to chromosomal deletions (aneuploidy) and introduction of abnormalities in the embryo. Implications processed by Cas9 are degenerative in gene editing, causing enforcement of genetic diseases.
Addressing this bioethical concern in this research can lead to improvements in the CRISPR-Cas 9 technology system by creating more accurate and precise methods of re-engineering the Cas 9 protein. This can be modified with sgRNA software as it provides a wider range of capabilities in cell development and genetic engineering studies to ensure the DNA is cutting the correct DNA sequence. Additionally, test uncertainty can be achieved to minimize false results from CRISPR-Cas9 systems, thus ensuring safety when using multiplexed systems.
CRISPR-Cas9 technology systems in genome editing are also being used in germline cells in humans. This can cause many ethical issues when performing gene editing since factors of morality and consent come into play. An example is the study conducted by (Cyranoski et al. 2015) in applications for gene editing in somatic cells. This was performed by a Chinese scientist named Huang on CRISPR-Cas9. Huang and his team saw ethical issues elucidating across germline cells and found that there are no therapeutic values to gene editing. In addition, there was a risk in the use of informed consent when using the applications of the CRISPR-Cas9 system. Huang studied single-cell fertilized human embryos that were created from vitro. This portrayed the embryos as containing an additional set of chromosomes (polyploid). Huang studied the HBB gene in the CRISPR-Cas9 system. The HBB gene expresses the human B-globin protein which is accountable for B-thalassaemia (Cyranoski et al. 2015). The embryos (86) were injected and held for two days, which allowed CRISPR-Cas9 to replace segments of missing DNA. Out of the surviving embryos, only 28 were genetically spliced properly, retaining the missing material. This raised the question about the non-surviving embryos. Huang found that this was due to off-target mutations introduced in the CRISPR-Cas9 system (Cyranoski et al. 2015). This raises ethical concerns since genome editing can be considered harmful and lead to lethal or harmful mutations, which are unintended. This can be passed onto future generations and not only harm the individual organism but hurt the individual’s offspring. In addition, ethical concerns can be seen in off-targeted mutations due to the abnormalities in an embryo. To decrease the number of off-target mutations, different models can be used by concentrating on enzymes to cleave and regulate the organisms lifespan before a mutation or DNA repair arises. Different methods and biological tools can effectively edit genes other than CRISPR-Cas9 such as TALEN (Transcription activator-like effector nuclease), which is more precise in gene editing. (Cyranoski et al. 2015).
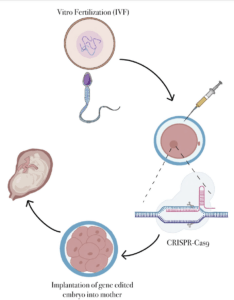
Currently, CRISPR-Cas9 is also being used in human clinical trials to test whether or not it is effective at editing DNA in the human body in order to treat diseases. Some diseases that are being tested with CRISPR-Cas9 are cancer, HIV, ALS, Cystic Fibrosis, etc. 12 . This situation raises ethical concerns because of the uncertainty of the CRISPR/Cas9 usage when attempting to treat these diseases. Since these trials may or may not be effective, the patients might develop a broad range of severe side effects. In most clinical trials, there is no way to receive unproven treatment. While some patients arrive to these trials during the early stages of their disease, other patients will start in later stages. All those who are arriving to the trials in later stages have a lower chance of living with the negatives of the trial since there would be a significant difference in the amount of information regarding side effects, toxicity, dosages, etc. 12 . Even though the intention of clinical trials is to improve someone’s life, sometimes there is no way for the clinical trial’s principal investigator to know whether or not the positive/negative effects are derived from CRISPR or not. Most of the patients that decide to be part of a clinical trial do it with the hopes that this treatment will help them recover or improve their health. However, as mentioned before, that is not always the case. When patients choose to receive CRISPR-Cas9 treatments, they are possibly risking their life and time if the treatment does not work. They are also exposing themselves to the possibility of other mutations arising since, as mentioned before, the effects of CRISPR on different patients are unpredictable at this time. There are many clinical trials testing CRISPR-Cas9 treatment in patients, but many of them have been terminated due to the side effects and dangers that come along with the treatment or that the treatment itself was not working and putting the patient’s life at risk by using CRISPR-Cas9 12 .
A gene therapy drug that worked but did not prevent long-term outcomes was Toferson for ALS patients who had an SOD1 mutation. The drug did reduce the amount of SOD1 protein in the cerebral spinal fluid and slightly slowed the progression of the disease in slower-progressing patients from the beginning, but it did not show any significant differences from the placebo group. 15 There are not a lot of treatments available for ALS, so for the patients who were slowly progressing, this treatment was worth the try. However, for the faster-progressing group, it may or may not have been worth trying because there are two other drugs for ALS that are proven to extend the lifetime of a person by a couple of months. 15 Although testing CRISPR-Cas9 in human subjects for diseases may seem unethical, sometimes clinical trials are the only way to test if this method works or not. In this case, the greater good is being a priority since all this is done in the hopes for the development of a solid new treatment that will help thousands of lives. Furthermore, there is an extensive list of steps, stages and tests that need to be done in order to even look at the possibility of having trials with human beings. These stages and testing could include, but are not limited to, cell testing and nonhuman animal testing.
Although, at any rate, the argument against CRISPR-Cas9 gene editing faces challenges, it can be readily answered through the use of editing strategies for medicinal purposes. Manipulating DNA is a powerful tool in biotechnology that can help researchers build a strong foundation for genetic diseases. An example from the study conducted by Vermersch et al. (2019) for gene editing strategies from the CRISPR-Cas9 system can be notably seen in embryonic stem cells. 17 An experiment was conducted on transgenic animals through microinjection for editing their genetic material through Cas9. The microinjection was placed in a zygote and then implanted into a genetically edited zygote. This allows scientists to then choose the organism from the edited genome and put it through the CRISPR-Cas9 system to correct any mutations or DNA repairments from cell division. Another experiment conducted by Khalaf et al. (2020) for CRISPR-Cas9 in Cancer Immunotherapy: Animal Models and Human Clinical Trials 18 CRISPR-Cas 9 uses modifications in genes to tag mutations in vivo and observe them in animals. Applications in CRISPR for cancer have become popularized due to providing in depth data and informative genetic biomarkers in embryonic stem cell research. Genetic engineering provides an important effect in cancer biology by providing gene screening and gene therapy. A study conducted by Li et al. (2020) with mice containing the adeno-associated viral mutations was detected for small cell lung carcinoma, which was transfected with a viral vector containing gRNA, which was used to tag the cancer. 19 CRISPR-Cas9 uses genetic screens in order to detect mutations and biomarkers for oncogenes that are associated with p53 and KRAS. CRISPR-Cas9 can be beneficial in precisely editing genomic DNA and cleaving mutant DNA in an organism for medicinal purposes. Not only is it important in removing viral vectors from DNA in bacteria and translocating immunotherapy in eukaryotes, but it is also important in genetic research. This is due to editing genomic sequences and changing the integrity of expression to restore broken ends.
CRISPR-Cas9 is a form of technology that is used for gene editing paving the way for new genomic research and gene-mediated efficiency. It has popularized its effect in genetic engineering due to its precision and affordability and has placed value in therapeutic effects. Although CRISPR-Cas9 has spread its efficiency in genome editing, a large application is processed in the bioethical community from its harmful effects. The effects are correlated in inaccuracy of technology, introduction of new mutations from Cas9’s influence and efficiency in trials. A major part of the application to CRISPR-Cas9 can go beyond gene editing. Improvements in the system need to specify in stopping off-targeting genes, precision in cleaving viral DNA and increasing specificity in sgRNA and Cas9.
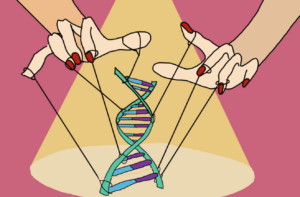
- Barman, A., Deb, B., & Chakraborty, S. (2019). A glance at genome editing with CRISPR–Cas9 technology. Current Genetics, 66(3), 447–462. https://doi.org/10.1007/s00294-019-01040-3 ↵
- Rodríguez‐Rodríguez, D. R., Ramírez‐Solís, R., Garza‐Elizondo, M. A., De Lourdes Garza‐Rodríguez, M., & Barrera-Saldaña, H. A. (2019). Genome editing: A perspective on the application of CRISPR/Cas9 to study human diseases (Review). International Journal of Molecular Medicine. https://doi.org/10.3892/ijmm.2019.4112 ↵
- Rodríguez‐Rodríguez, D. R., Ramírez‐Solís, R., Garza‐Elizondo, M. A., De Lourdes Garza‐Rodríguez, M., & Barrera-Saldaña, H. A. (2019). Genome editing: A perspective on the application of CRISPR/Cas9 to study human diseases (Review). International Journal of Molecular Medicine. https://doi.org/10.3892/ijmm.2019.4112 ↵
- Cheng, H., Zhang, F., & Ding, Y. (2021). CRISPR/CAS9 delivery System engineering for genome editing in therapeutic applications. Pharmaceutics, 13(10), 1649. https://doi.org/10.3390/pharmaceutics13101649 ↵
- Zuccaro, M. V., Xu, J., Mitchell, C. A., Marín, D., Zimmerman, R. A., Rana, B., Weinstein, E., King, R. T., Palmerola, K. L., Smith, M. E., Tsang, S. H., Goland, R., Jasin, M., Løbo, R. A., Treff, N. R., & Egli, D. (2020). Allele-Specific Chromosome Removal after Cas9 Cleavage in Human Embryos. Cell, 183(6), 1650-1664.e15. https://doi.org/10.1016/j.cell.2020.10.025 ↵
- Zuccaro, M. V., Xu, J., Mitchell, C. A., Marín, D., Zimmerman, R. A., Rana, B., Weinstein, E., King, R. T., Palmerola, K. L., Smith, M. E., Tsang, S. H., Goland, R., Jasin, M., Løbo, R. A., Treff, N. R., & Egli, D.(2020). Allele-Specific Chromosome Removal after Cas9 Cleavage in Human Embryos. Cell, 183(6), 1650-1664.e15. https://doi.org/10.1016/j.cell.2020.10.025 ↵
- Zuccaro, M. V., Xu, J., Mitchell, C. A., Marín, D., Zimmerman, R. A., Rana, B., Weinstein, E., King, R. T., Palmerola, K. L., Smith, M. E., Tsang, S. H., Goland, R., Jasin, M., Løbo, R. A., Treff, N. R., & Egli, D. (2020). Allele-Specific Chromosome Removal after Cas9 Cleavage in Human Embryos. Cell, 183(6), 1650-1664.e15. https://doi.org/10.1016/j.cell.2020.10.025 ↵
- Zuccaro, M. V., Xu, J., Mitchell, C. A., Marín, D., Zimmerman, R. A., Rana, B., Weinstein, E., King, R. T., Palmerola, K. L., Smith, M. E., Tsang, S. H., Goland, R., Jasin, M., Løbo, R. A., Treff, N. R., & Egli, D. (2020). Allele-Specific Chromosome Removal after Cas9 Cleavage in Human Embryos. Cell, 183(6), 1650-1664.e15. https://doi.org/10.1016/j.cell.2020.10.025 ↵
- Zuccaro, M. V., Xu, J., Mitchell, C. A., Marín, D., Zimmerman, R. A., Rana, B., Weinstein, E., King, R. T., Palmerola, K. L., Smith, M. E., Tsang, S. H., Goland, R., Jasin, M., Løbo, R. A., Treff, N. R., & Egli, D. (2020). Allele-Specific Chromosome Removal after Cas9 Cleavage in Human Embryos. Cell, 183(6), 1650-1664.e15. https://doi.org/10.1016/j.cell.2020.10.025 ↵
- Zuccaro, M. V., Xu, J., Mitchell, C. A., Marín, D., Zimmerman, R. A., Rana, B., Weinstein, E., King, R. T., Palmerola, K. L., Smith, M. E., Tsang, S. H., Goland, R., Jasin, M., Løbo, R. A., Treff, N. R., & Egli, D. (2020). Allele-Specific Chromosome Removal after Cas9 Cleavage in Human Embryos. Cell, 183(6), 1650-1664.e15. https://doi.org/10.1016/j.cell.2020.10.025 ↵
- Zuccaro, M. V., Xu, J., Mitchell, C. A., Marín, D., Zimmerman, R. A., Rana, B., Weinstein, E., King, R. T., Palmerola, K. L., Smith, M. E., Tsang, S. H., Goland, R., Jasin, M., Løbo, R. A., Treff, N. R., & Egli, D. (2020). Allele-Specific Chromosome Removal after Cas9 Cleavage in Human Embryos. Cell, 183(6), 1650-1664.e15. https://doi.org/10.1016/j.cell.2020.10.025 ↵
- Brokowski, C., & Adli, M. (2019). CRISPR Ethics: moral considerations for applications of a powerful tool. Journal of Molecular Biology, 431(1), 88–101. https://doi.org/10.1016/j.jmb.2018.05.044 ↵
- Brokowski, C., & Adli, M. (2019). CRISPR Ethics: moral considerations for applications of a powerful tool. Journal of Molecular Biology, 431(1), 88–101. https://doi.org/10.1016/j.jmb.2018.05.044 ↵
- Brokowski, C., & Adli, M. (2019). CRISPR Ethics: moral considerations for applications of a powerful tool. Journal of Molecular Biology, 431(1), 88–101. https://doi.org/10.1016/j.jmb.2018.05.044 ↵
- Miller, T. M., Cudkowicz, M., Genge, A., Shaw, P. J., Sobue, G., Bucelli, R. C., Chiò, A., Van Damme, P., Ludolph, A. C., Glass, J. D., Andrews, J., Babu, S., Benatar, M., McDermott, C. J., Cochrane, T., Chary, S., Chew, S., Zhu, H., Wu, F., . . . Fradette, S. (2022). Trial of Antisense Oligonucleotide Tofersen for SOD1 ALS. The New England Journal of Medicine, 387(12), 1099–1110. https://doi.org/10.1056/nejmoa2204705 ↵
- Miller, T. M., Cudkowicz, M., Genge, A., Shaw, P. J., Sobue, G., Bucelli, R. C., Chiò, A., Van Damme, P., Ludolph, A. C., Glass, J. D., Andrews, J., Babu, S., Benatar, M., McDermott, C. J., Cochrane, T., Chary, S., Chew, S., Zhu, H., Wu, F., . . . Fradette, S. (2022). Trial of Antisense Oligonucleotide Tofersen for SOD1 ALS. The New England Journal of Medicine, 387(12), 1099–1110. https://doi.org/10.1056/nejmoa2204705 ↵
- Vermersch, E., Jouve, C., & Hulot, J. (2019). CRISPR/Cas9 gene-editing strategies in cardiovascular cells. Cardiovascular Research, 116(5), 894–907. https://doi.org/10.1093/cvr/cvz250 ↵
- Khalaf, K., Janowicz, K., Dyszkiewicz-Konwińska, M., Hutchings, G., Dompe, C., Moncrieff, L., Jankowski, M., Machnik, M., Oleksiewicz, U., Kocherova, I., Petitte, J., Mozdziak, P., Shibli, J. A., Iżycki, D., Józkowiak, M., Piotrowska‐Kempisty, H., Skowroński, M. T., Antosik, P., & Kempisty, B. (2020). CRISPR/CAS9 in cancer immunotherapy: animal models and human clinical trials. Genes, 11(8), 921. https://doi.org/10.3390/genes11080921 ↵
- Li, H., Yang, Y., Hong, W., Huang, M., Wu, M., & Zhao, X. (2020). Applications of genome editing technology in the targeted therapy of human diseases: mechanisms, advances and prospects. Signal Transduction and Targeted Therapy, 5(1). https://doi.org/10.1038/s41392-019-0089-y ↵
37 comments
Michael Rodriguez
Great and educated insight!
Tanya Carrasco
great work!!
Salma Gutierrez
amazing work
Alexandra Aguilar
Wow that’s really really really good
Daphne Aguilar
Great work!
joseph suess
Outstanding work really helped me learn a lot
erick
good job!
Christian
Wow, this was the most helpful website I’ve found. Greatly structured, very detailed and informational. Thank you
Alanna Crites
Brilliant and fascinating work!
Lauren Randel
Really good job!!